Education Series
Number 5.2
February 2001 (Revised)
Joycelyn Woods has a graduate degree in neuroscience and psychopharmacology. She has published in neuroscience journals and is recognized internationally for her methadone advocacy work. She is a recipient of the “Richard Lane Methadone Advocacy Award.”
Basic Opioid Pharmacology
All natural and synthetic opioids exhibit a three dimensional T-shaped
configuration (Barchas, Berger, Ciaranello and Elliott, 1977). This T-shaped
molecule has two broad hydrophobic surfaces which are at right angles and a
methylated nitrogen which is usually charged at physiological pH. The charged
nitrogen is essential for activity and lies in one of the hydrophobic planes.
A hydroxyl group at carbon 3 on the other plane is also essential. This
configuration which all opioids have is called the piperidine
ring. Figure 1 is the structure of morphine with the piperidine
ring indicated by bold lines. Simple changes on the morphine molecule produces
several semisynthetic derivatives. Diacetylmorphine, or heroin is made from
the morphine molecule by the acetylation of derivatives of the natural opium
alkaloids, there are a number of other structurally distinct chemical classes
of both the phenolic and the alcoholic OH groups (see Table 1). In addition to
morphine, codeine and the semisynthetic drugs with pharmacological actions
similar to those of morphine (Gilman, Rail, Niles and Taylor, 1990). These
groups although diverse share commonalties including the capacity to produce
analgesia, respiratory depression, gastrointestinal spasm, and morphine-like
physical dependence.
These compounds include the morphinians, benzomorphans, methadones,
phenylpiperidines and propionanilides. While two dimensional representatives
of the compounds appear to be quite different, three dimensional molecular
models show certain common characteristics.
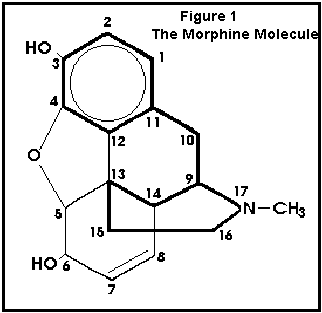
|
Figure 1
The Morphine Molecule.
The structure of morphine and all opium derivatives are characterized by the
piperdine ring which is indicated with bold lines. From Gilman, Rail, Niles,
Taylor, Goodman and Gilman’s The Pharmacological Basis of Therapeutics (1990).
|
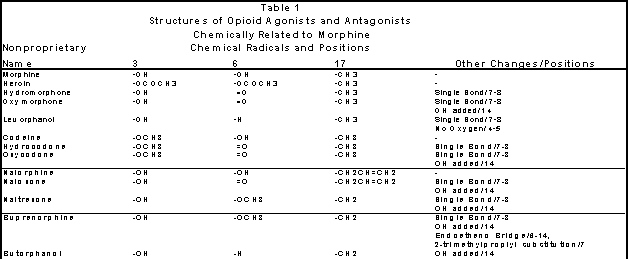
Table 1. Structures of Opioid Agonists and Antagonists Chemically Related to Morphine.
Simple changes at positions 3, 6 and 17 of the morphine molecule (see Figure 1) can create dramatic
changes in the action of a compound. From Gilman, Rail, Niles, Taylor, Goodman and Gilman’s The Pharmacological Basis of Therapeutics (1990).
|
Endogenous Opioids
The term endorphin is used to characterize a group of endogenous peptides whose
pharmacological action mimics that of opium and its analogs (Gilman, Rail, Niles
and Taylor, 1990). The endogenous opioid system is complex with a multiplicity
of functions within any given organism (Goldstein, 1994). There exists about two
dozen known endogenous opioids which belong to one of three endogenous opioid
systems: 1) the endorphin system, 2) the enkephalins, and 3) the dynorphin
system.
The endogenous opioid system may play a role in a wide
variety functions such as, the production of analgesia, attention, memory,
catatonia, schizophrenia, manic depression, immune function, endocrine function,
appetite regulation, sexual behavior, postpartum depression, release of several hormones, locomotor activity,
anticonvulsant activity, body temperature regulation, meiosis (pin point
pupils), shock from trauma, respiration, sleep, drug dependence, anxiety,
stress, mood and behavior (Gilman, Rail, Niles and Taylor, 1990; Goldstein,
1994).
Endorphins are peptides. A peptide is a biologically active substance composed of amino
acids that are produced in neurons. Today peptides are considered to be a
distinct and separate group of psychoactive substances in the brain (Goldstein,
1994).
The Target of Action: The Receptor
Most psychoactive drugs exert their action at a receptor. This can be thought of as a
“lock and key” with the key as the drug opening the lock, or receptor.
Opiate receptors can be broken down further into types: the m (mu) receptor
prefers morphine, heroin and methadone, the e (epsilon) receptor prefers
b-endorphin (beta-endorphin), the d (delta) receptor prefers enkephalins, and
the k (kappa) receptor that prefers dynorphins (Goldstein, 1994). Some receptors
are broken down further into subtypes as in the k1 and k2 receptors. A substance
that binds to a receptor is called a ligand, thus endorphins are the natural
ligand for the opiate receptor. The entire endogenous opioid system is referred
to as the “Endogenous Opiate Receptor Ligand System”.
Receptors have several properties. Any substance, including the endogenous ligand or any
exogenous compound that attaches to a receptor occurs through a process
of chemical bonding (Goldstein, 1994; Pratt and Taylor, 1990). This kind of
binding to a receptor is referred to as specific. Affinity refers to the
strength that a substance binds to a receptor. Some chemical bonds are stronger
than others resulting in some substances having a greater affinity than others
for a receptor. In respect to opiate receptors and opioid analgesics the
stronger the affinity, the stronger the analgesic properties of the substance.
Therefore, morphine which is a strong analgesic has a stronger affinity for the
opiate receptor than codeine which is a weaker analgesic. Opiate receptors have
been found in every vertebrate and even in some invertebrate species. Therefore,
opiate receptors and the endogenous opioids are basic within the scheme
of evolution. Their vast distribution in species implies that
endorphins were important in the scheme of evolution, and particularly mammalian
(Goldstein, 1994).
Agonists and Antagonists
An agonist is a substance that binds to the receptor and produces a response that
is similar in effect to the natural ligand. In contrast, antagonists bind to the
receptor but block it by not allowing the natural ligand or any other compound
to bind to the receptor.
Antagonists do not cause the opposite effect. They merely fit into the receptor and block
any other substance from binding to it. For example, narcotic antagonists such
as naloxone or its’ predecessor Naline are administered to reverse a heroin or
opioid overdose. This is achieved because opioid antagonists have a greater
affinity for the opiate receptor than agonists and in fact the affinity is so
strong that narcotic antagonists can literally knock an agonist right out of the
receptor. The effect is very fast and the overdose victim will wake up within
minutes, or seconds even. Individuals dependent on heroin, or other opioids such
as methadone can wake up in withdrawal.
Heroin, methadone and morphine are opioid agonists. Narcotic antagonists are produced by
a change on the nitrogen atom of an opioid agonist. Thus nalorphine is produced
from a change in the nitrogen atom of the morphine molecule and naloxone is
produced from oxymorphone (see Table 1). Naltrexone is a long acting narcotic
antagonist which is used for maintenance treatment. It works by binding to the
receptor over a 24 hour period thus making any injection or administration of an
opioid agonist ineffective. It must be emphasized that naltrexone does not have
agonist properties it merely blocks every opiate receptor irrespective of that
receptors function. Thus, long term treatment with narcotic antagonists can also
block important biological functions and various side effects have been
reported, including hypersexuality.
Methadone and Congeners
Germany has been a leader in the discovery and production of pharmaceuticals since the
mid-Nineteenth Century. In the 1850s German scientists discovered the first
molecular structure of a substance, which was morphine. In the 1930s scientists at I.G. Farbenindustrie (Hoechst-Am-Main) were searching for an
analgesic that would be easier to use during surgery and also have low addiction
potential.
In 1937 Max BockmÜhl and Gustav Ehrhart discovered a
synthetic substance they called Hoechst 10820 or polamidon and whose structure
had no relation to morphone or the opioid alkaloids (BockmÜhl and Ehrhart,
1949). On September 11, 1941 BockmÜhl and Ehrhart filed an application for a patent (see Figure 2).
At the end of WW2 the town of Hoechst was occupied and the patients of I.G. Farbenindustrie
became property of the U.S. Hoechst 10820 was named ‘methadon’ and taken to
the Public Health Service Narcotic Treatment Center at Lexington, KY.
Figure 2. Methadone Patent. The Original Patent for Methadone. (Note: This figure was scanned from a poor copy and in order to make it readable text was entered that
could be incorrect.)
|

|
Research was conducted in which addicts where found to respond favorably
to it and thus methadone was adopted to withdraw addicts from narcotics (Isbell,
Wikler and Eddy, 1947). However, methadone’s properties as a maintenance medication for addicts was not
realized. For the next two decades the primary use of methadone was in withdrawing addicts
from narcotics. In the early 1960s Dr. Dole, a metabolic specialist at The
Rockefeller University and Dr. Marie Nyswander, a psychiatrist that specialized
in addiction (Dr. Nyswander could easily be called the first Addiction
Specialist) began research to find a medication that could be used to maintain
addicts. At the start of their research they theorized that addicts would be
better if they could be prescribed a medication instead of purchasing unknown
substance on the illicit market (Dole, 1988).
Unfortunately their first trial with morphine seemed a failure because their subjects were
still occupied with obtaining their drugs. Since the standard medication to
withdraw addicts was methadone they switched their subjects over to it in
preparation to end the research. However, in an attempt to have something to
show for their work they decided to increase the methadone dose and run the same
tests on their subjects before discharging them from the hospital ward (Anon,
1994). And then something happened! Their subjects stopped sitting in front of the television waiting for the next
injection; one subject asked that he be allowed to leave the ward to go to work,
one who had never completed high school now also wanted to leave the ward to
return to school, and an other began painting — their subjects began to act
like normal people with interests in things other than drugs.
Dole nand Nyswander soon found that once an adequate treatment dose was reached that
their subjects could be maintained with out needing increases for a prolonged
period of time. Unlike morphine, their subjects on methadone did not need
increasing doses in order to achieve the same effect. They realized that they
had found a maintenance medication.
Dole and Nyswander underwent another transformation during their initial research.
From their observations they began to postulate that opiate addiction was a
metabolic disease and like the diabetic needing insulin, addicts needed
methadone to maintain normal functions (Dole, 1988). Their ideas were radical
and the Bureau of Narcotics (BON, now the DEA) was threatened by them. The BON
informed Dr. Dole that he was breaking the law and that they would stop his
research unless he ceased it himself. At this point Dr. Dole told a very brave
stance. After obtaining legal advise that their work at The Rockefeller
University was perfectly legal Dr. Dole invited the BON to go ahead and
prosecute him. He also informed the BON that prosecution would create a proper
ruling on the matter. The BON backed down or at least ceased their overt threats
to the project.
Before we go further lets clear up another myth. Methadone, or Dolophine was not named
after Adolph Hitler. The “dol” in Dolophine comes from the Latin root
“dolor.” The female name Dolores is also derived from it and the term
dol is used in pain research to measure pain e.g., one dol is 1 unit of pain.
Dolophine is the American trade name given to methadone by Eli Lilly during the
1950’s.
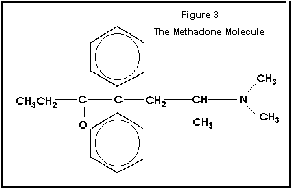
|
Figure 3 The Methadone Molecule.
The two dimensional representation of the methadone
molecule is very different from that of morphine. However, molecules are three
dimensional and the methadone molecule bends into a structure that is very
similar to morphine and the piperdine ring. The is probably how methadone is able to fit into the opiate
receptor. From Gilman, Rail, Niles, Taylor, Goodman and Gilman’s The
Pharmacological Basis of Therapeutics (1990).
|
Methadone looks strikingly different from other opioid agonists, however it has steric
forces which produce a configuration that closely resembles that of other
opiates (Figure 3). In other words, steric forces Bend the molecule of methadone
into the correct configuration to fit into the opiate receptor.
|
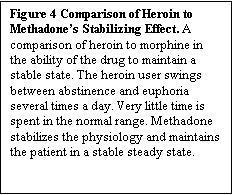
|
Figure 4. Comparison of Heroin to Methadone’s Stabilizing Effect. A comparison of heroin to morphine in the ability of the drug to maintain a stable state. The heroin user swings between abstinence and euphoria several times a day. Very little time is spent in the normal range. Methadone stabilizes the physiology and maintains the patient in a stable steady state.
|
How Methadone Works Its Miracle
Methadone has a long half life in comparison to other opiates averaging about 28 hours and
is active without first passing through the liver. As the dose is increased over
time excess methadone is stored in body tissue and blood stream. This is how
methadone works its ‘time release trick’ and can last for 24 hours or more (Inturrisi
and Verebey, 1972). The higher the dose the more that is stored. As
stabilization is reached so the patient is in a steady state then narcotic
blockade is achieved. Basically narcotic blockade is tolerance but with special
properties. A patient at narcotic blockade will not experience drug craving and
they are also protected from overdose should they attempt to take an illicit
drug or opiate that was not prescribed.
Once in the blood stream methadone is slowly passed to the brain when it is needed to
fill opiate receptors. Methadone has a higher affinity for the opiate receptor
than other opiates. Thus, the long half life along with storage and methadones
high affinity for the opiate receptor creates a steady state and the narcotic
blockade (see Figure 4). This is why some patients on blockade doses (70 mg/day
or more) are able to go for a day or two without their medication. Of course the
down side to this is that when a patient misses a dose they will begin to
“destabilize” which places them at risk of overdose should they attempt
to administer other opiates. They are slowly loosing the narcotic blockade and
may begin to experience drug hunger and craving. No other medication has
received the scrutiny and evaluations that methadone has which continue to this
day (over thirty years) (Ball and Ross, 1991; Caplehorn, 1994; Cooper, 1992;
Dole, 1988; Dole and Joseph, 1978; Dole and Nyswander, 1965; GAO, 1990; Gearing
and Schweitzer, 1974; Joseph and Dole, 1970; Zweben and Payte, 1990).
Methadone is perhaps one of the safest drugs known with only a few side effects which
usually subside after stabilization or adjustment of dose during the first year of
treatment. There are no reports of anyone being allergic to methadone.
The current theory of why methadone delays the onset of opiate abstinence syndrome
for 24-48 hours, but is only effective for pain relief for 4-6 hours, appears to
be because these two phenomena involve two different part s of the brain each
with slightly different m receptors. Pleasure, and much of the pain relief
associated with opiates, occurs in the Nucleus Accumbens (NA) and the Ventral
Tegmental Area (VTA); however, withdrawal appears to be localized in the
Periaquaductal Gray (PAG). It appears that some of the products of methadone
biotransformation bind better to the PAG than in the VTA and NA. The phenomenon
is responsible for methadone’s ability to delay the onset of withdrawal for
extended periods of time.
When a drug is in your bloodstream, it is not always just a free drug roaming around
waiting to interact with a receptor. Virtually all drugs, depending on their lipophilicity (attraction to lipids or fat) and
hydrophilicity (attraction to water), bind to some extent with plasma (blood)
proteins. Recall that when a ligand or drug binds to a receptor this kind of binding is called specific.
The binding to a receptor occurs because of specific chemical bonds and
the strength of the bonding depends of the affinity. Chemical bonds are common
to all substances whether your body produces the substance or it is a drug that
you take. In this way substances can attach to cell membranes or plasma
proteins but the binding is not very strong. This kind of binding is called non specific.
And in fact this was the fact that Candice Pert solved when she located the opiate
receptor (Pert and Snyder, 1973). Both Dole and Goldstein could not
differentiate between specific binding to a receptor and non specific binding to
membranes (Ingolia and Dole, 1970). In simple terms Pert theorized that she should be able to wash away the non
specific binding because the bonds are not strong. And that was what she did, after applying the radioactive opiate drug to
the tissue sample, she washed it. What was left was specific binding to the
opiate receptor.
When methadone is bound to the plasma proteins it kind of works like a time release
capsule. The methadone is kept in the blood stream until you need it. Typically methadone is almost 80% bound to
plasma proteins. However, since t his binding is non specific the bond can be
easily broken which releases the methadone. The methadone is then free to interact with the receptor.
Bioavailability
Drugs that are taken orally do not always get completely in to the blood stream like a
drug taken intravenously (see Part1, Administration). Many are poorly absorbed
when taken orally, like methadone. Heroin and morphine are about 85-90%
inactivated by the liver before getting into the blood stream. This is known as
the “first-pass effect.” Methadone has an average bioavailability of 0.5 or
50%, which means that half of the methadone gets into the blood stream and half
of it just passes through the GI tract, without being utilized. Many things can influence the bioavailability and of the
major influences it is the pH. Food that have eaten can change your
pH to acidic or basic (sugar). When methadone is in an acid environment, much
less of it gets absorbed. This means that a glass of juice can
hinder absorption (acidic) and an anti-ulcer medication can increase absorption
(basic).
Bioavailability:
The Mystery of the Diskette vs Liquid!
Many patients will swear that the diskettes (biscuits) are stronger and last longer
that the liquid methadone. The diskettes are designed to stay bound to an insoluble matrix until the acid in
your stomach hydrolyzes it (frees it). Thus, the insoluble matrix helps to keep methadone around longer in your stomach, in
comparison to the liquid version which could pass right through without being
used depending upon your pH. Also, eating before dosing can definitely decrease bioavailability, whereas eating
right after a dose appears to increase it.
Many people in treatment think that the powder at the bottom is talc.
It is not; it is the actual methadone bound to the matrix.
Methadone Serum Levels (MSL):
Helpful Tool or Malevolence Science
In the past ten years the ability to measure the level of methadone in the blood
has become available. MSLs have been more useful in helping clinicians
understand methadone metabolism rather than as a clinical tool. Methadone blood levels are measured in nanograms per
milliliter (ng/mL). After taking a dose MSLs will “peak” at 3 to 4 hours followed by a slow decline over the
next 24 hours or “trough”. Researchers have confirmed an MSL of 150-600 ng/mL in order to suppress drug craving and a
trough level above 400 ng/mL to achieve narcotic blockade (Dole, 1988; Payte and Khuri, 1992).
Unfortunately measuring MSLs only tells the clinician what is occurring at the time that the
blood sample was taken. Attempting to take peak and tough levels can be
intrusive to the patient, costly and unnecessarily time consuming. Recent
studies have found no correlation between a patient’s dose and MSL and that a
group of patients taking the same methadone dose can vary significantly
(Bradbury and Paris 1998). Thus MSLs are more useful in helping the clinician to confirm inadequate
doses that for determining optimum dose (Leavitt, Shinderman, Maxwell,
Eap and Paris, 2000).
Unfortunately many clinicians have begun to use MSL as an excuse to withhold an adequate dose
from patients. Some clinics now require that a patient have blood levels done
before they can get an increase instead of relying on patient reports, continued
opiate use, clinical observation and expertise. Thus often patients have to wait
for weeks for an increase because they must make an appointment to have blood
levels done and then wait for the laboratory results. And since blood levels do not tell the full story of what may be occuring
some patients may have normal MSLs and yet still experience abstinence symptoms.
Typically these patients are denied their much needed increase.
Dole (1988) has stressed that the use of MSLs are not necessary and that adequate
dosing can be achieved by “listening and evaluating the patient’s report”
along with other clinical variables.
References
Anon (1994). Conversation with Vincent Dole. Addiction 89: 23–9.
Ball, J.C. and Ross, A. (1994). The Effectiveness of Methadone Maintenance Treatment. New York: Springer-Verlag.
Barchas, J.D., Berger, P.A., Ciaranello, R.D. and Elliot, G.R. (1977). Psychopharmacology. From Theory to Practice. New York: Oxford University Press.
Download PDF 
Next Education Series
Index Education Series
|